Evaluation of the Renomination of Radionuclides as Chemicals of Mutual Concern Under the Great Lakes Water Quality Agreement
Executive summary
The Great Lakes Water Quality Agreement (GLWQA) is an agreement between Canada and the United States of America, which includes Annex 3: “Chemicals of Mutual Concern (CMCs)” and allows stakeholders to propose the designation of specific chemicals as CMCs. Following this process, in March 2016, radionuclides were nominated as a candidate CMC by the Canadian Environmental Law Association (CELA), and the nomination was supported by 110 environmental, health and advocacy groups across the U.S. and Canada, including the Great Lakes Indian Fish & Wildlife Commission, which represents 11 tribal governments in the U.S. In 2022, CELA renominated radionuclides as CMCs, applying the newly developed binational screening criteria.
Environment and Climate Change Canada (ECCC) is the Canadian decision-making authority regarding the designation of CMCs. Recognizing the Canadian Nuclear Safety Commission’s (CNSC) regulatory responsibility as well as technical and scientific expertise on radionuclides, ECCC requested that the CNSC review CELA’s renomination and provide advice with respect to ECCC’s pending decision.
This report summarizes the CNSC’s assessment of the renomination, focussing on whether any new information is presented that was not addressed in the CNSC’s assessment (CNSC 2018) of the initial CELA nomination. The CNSC CMC assessment (2018) determined that there is no evidence to indicate that radionuclides currently within the Great Lakes are posing an unreasonable risk to the environment or human health and safety. Moreover, it was concluded that existing regulatory processes, risk management activities, and strong intergovernmental relationships ensure the safe management of radionuclides.
Once again, the CNSC has been asked to review the renomination (CELA and TFGLBN 2022). In light of the CNSC’s detailed review and reporting in the previous CMC assessment (CNSC 2018), this review, for the most part, limits itself to directing readers to the relevant sections of the previous CNSC CMC assessment for more detailed responses with additional information or commentary limited to addressing statements in the renomination report that merit correction or clarification.
This review also provides the opportunity to update interested parties on developments since completion of the previous CNSC CMC assessment. The results of ongoing monitoring programs and risk assessments, including significant improvements with respect to making such information more readily available to the public, are summarized. An update is also provided on the continued delivery of radiation and radionuclide research in Canada, with a focus on environmental radioactivity and the Great Lakes where relevant.
The collection of evidence shows that the conclusions drawn in the CNSC CMC assessment (2018) continue to remain valid and that radionuclides within the Great Lakes do not pose an unreasonable risk to public health or the environment. Therefore, the report concludes that radionuclides are not recommended as a candidate CMC for further evaluation under Annex 3 of the GLWQA.
Table of Contents
- Evaluation of the Renomination of Radionuclides as Chemicals of Mutual Concern Under the Great Lakes Water Quality Agreement
- Executive summary
- 1. Introduction
-
2. Review of the renomination following binational screening criteria
- 2.1 Toxic: Is the chemical substance toxic, persistent, and/or bioaccumulative?
- 2.2 Release: To what extent is the chemical substance released in the Great Lakes basin?
- 2.3 Levels: Are levels of the chemical substance harmful, or likely to become harmful, in the Great Lakes environment?
- 2.4 Route of exposure: Are the Great Lakes a route of exposure to humans or wildlife for this chemical substance, and are impacts or likely impacts caused by routes of exposure?
- 2.5 Scale: Does the geographic scale of the levels of the chemical substance in the Great Lakes have binational significance?
- 2.6 Management: To what extent are the uses and releases of the chemical substance controlled/managed?
- 3. Renomination comments on the 2018 Canadian Nuclear Safety Commission chemicals of mutual concern assessment
- 4. Conclusions and recommendations
- 5. References
- Appendix A. Updated data tables and figures
1. Introduction
Environment and Climate Change Canada (ECCC) is the Canadian decision-making authority as to whether radionuclides are to be considered as Chemicals of Mutual Concern (CMC) under the Great Lakes Water Quality Agreement (GLWQA). Recognizing the Canadian Nuclear Safety Commission’s (CNSC) regulatory responsibility as well as technical and scientific expertise on radionuclides, ECCC requested that CNSC review a non-governmental organization (NGO) nomination (CELA 2016) and provide advice with respect to ECCC’s pending decision.
In the absence of any formal screening criteria for identifying CMCs at the time of the initial review request, the CNSC published a review in 2018 that summarized the current state of science related to the environmental and health effects of radiation, outlined the historical and current data on radionuclides in the Great Lakes with an evaluation of the associated risks, and documented the international and national science and regulatory frameworks applicable to nuclear facilities and activities. The conclusions of the CNSC CMC assessment (CNSC 2018) are provided below. The full report is available open-access for download from the Great Lakes Binational Website (external).
“The data on the quantities of radionuclides monitored within the Great Lakes have been summarized and assessed using internationally and nationally recognized environmental and human health risk assessment approaches. The results are compared to science-based benchmarks for protection of the environment and of human health. This assessment, using the large volume of available environmental data and the best science on the effects of radiation on human health and the environment, indicates that there is no evidence to suggest that radionuclides pose an unreasonable risk to environment, health or safety within the Great Lakes basin ecosystem.
Furthermore, radionuclides are among the most heavily regulated substances both internationally and nationally. Canada has an independent national nuclear regulatory body (i.e., the CNSC), the mandate of which is to protect the environment, and the health and safety of persons. The CNSC has no promotional role for the industry. Strong inter-governmental relationships have been established both federally and provincially to ensure radionuclides and radiation are safely managed.”
An evaluation of the nomination was put on hold while Canada and the U.S. worked together to develop a set of binational screening criteria to provide a consistent framework for reviewing nominated CMCs. In 2021, formal binational screening criteria, a guidance document for nominations, and an example nomination were published on the Great Lakes water quality agreement website on binational screening criteria for nominated chemicals of mutual concern (external) in order to clarify and streamline the nomination process. Groups that had previously submitted nominations were afforded the opportunity for renomination incorporating the new criteria and guidance. Radionuclides were renominated by NGOs through this process (CELA and TFGLBN 2022).
This publication presents the CNSC’s consideration of the renomination of radionuclides as CMCs under the GLWQA. Due to the comprehensive nature of the previous CNSC CMC assessment (CNSC 2018), assessment of the renomination will focus on the following items:
- Consideration of any new scientific knowledge, facts, or information sources
- An update on new developments relevant to the nomination since the publication of the CNSC CMC assessment (CNSC 2018)
- Discussion of incorrect information or statements within the renomination (CELA and TFGLBN 2022)
For this purpose, this report is structured following the binational guidance document for Annex 3 chemical nominations and specified binational screening criteria (external) as applied in the renomination under review.
For completeness and easy reference, Table 1 below shows applicable sections in this report and the previous CNSC CMC assessment (2018) for each of the binational screening criteria.
Table 1: Binational screening criteria and applicable report sections.
Screening criterion | Section in this report | Applicable sections in CNSC CMC assessment (2018) |
---|---|---|
Toxic | 2.1 | 2.1, 2.2, 2.3, 2.3.1, 2.3.3, 4.2 |
Release | 2.2 | 3.0, 3.1, 3.2, 3.2.1, 3.2.2, 3.2.3, 3.2.4, 3.2.5, 3.2.6, 3.2.7, 3.2.8 |
Levels | 2.3 | 2.3, 2.3.1, 2.3.3, 3.0, 3.1, 3.2.4, 4.1, 4.2, 5.2.2 |
Route of exposure | 2.4 | 4.0, 4.1, 4.2, 4.2.1, 4.2.2, Appendix A, B, C |
Scale | 2.5 | 3.1, 5.2 |
Management | 2.6 | 2.1, 2.3, 2.3.2, 5.0, 5.1, 5.2, 5.2.1, 5.2.2, 5.3, 5.3.1, 5.3.2, 5.3.3, 5.3.5 |
2. Review of the renomination following binational screening criteria
2.1 Toxic: Is the chemical substance toxic, persistent, and/or bioaccumulative?
One of the basic principles of toxicology is that “the dose makes the poison” (dosis sola facit venenum). This recognizes the fact that the probability of a substance producing a harmful biological effect is related to the quantity or dose to which biological organisms are exposed. Thus, extremely common substances, even those necessary to life such as water and oxygen, are toxic if an organism is exposed to sufficiently high doses. As a result, the listing of health effects that can result from exposure to sufficiently high quantities of a substance is of limited value when determining whether substances pose a health risk in real-world scenarios.
2.1.1 Has the chemical substance been found to be toxic?
It is an accepted fact that radiation doses of sufficient magnitude and/or for sufficient exposure periods can be detrimental to human and ecological health. What is relevant from a risk assessment and management perspective is whether doses arising from publicly beneficial activities pose an unreasonable risk to the environment. The binational guidance specifically addresses this, identifying assessments completed under the Canadian Environmental Protection Act (CEPA) as an example of acceptable assessments for the determination of “toxic.”
The renomination makes specific mention of the International Nuclear Workers Study (INWORKS) with respect to low-dose radiation health effects (Leuraud et al. 2015; Richardson et al. 2015; Thierry-Chef et al. 2015; Hamra et al. 2016; Gilles et al. 2017; Laurier et al. 2017; Richardson et al. 2018; Leuraud et al. 2021). INWORKS has been important to statistically refining low-dose radiation risk estimates based on extensive exposure and health monitoring requirements incorporated within the international radiation protection framework. This refinement is especially important with respect to the low dose exposure ranges associated with the nuclear sector. The INWORKS findings strongly support the current weight of evidence on radiation risk and the international radiation protection framework on which our regulatory dose limits are based (e.g., Linear-Non-Threshold model: additional information in CNSC 2018, section 2.3.1; Leblanc and Burtt 2019).
In fact, INWORKS is just one example of the extensive international network of science and research associated with radiation exposure upon which the international and national (CNSC and Health Canada) regulatory frameworks are founded (CNSC 2018, section 2.3; Leblanc and Burtt 2019) and against which they are continuously reviewed and updated. This extensive scientific and regulatory network exists because of the recognized importance of safety integration to the nuclear sector for a modern society with respect to science, technology, energy production, and medical applications.
As outlined in the CNSC CMC assessment (CNSC 2018, section 4.2) radionuclides have already been assessed under the CEPA, with respect to both ionizing radiation and chemical toxicity. The CEPA assessment concluded that ionizing radiation emitted by radionuclides released from licensed nuclear activities “are not entering the environment in quantities or concentrations or under conditions that have or may have an immediate or long-term harmful effect on the environment or its biological diversity” (ECCC and HC 2006). The assessment concluded that uranium as a chemical substance (i.e., acting as a heavy metal), released from uranium mines and mills, was CEPA toxic based on three sites in northern Saskatchewan (ECCC and HC 2006). Risk management activities were identified and implemented such that releases of uranium as a chemical toxicant no longer meet the classification as CEPA toxic (CNSC and EC 2009). In conclusion, radionuclides do not meet the current definition of “CEPA toxic” under the CEPA.
2.1.2 Is the chemical substance persistent and/or bioaccumulative?
In terms of persistence, section 2.1 “Basics of Radiation Science” of the CNSC CMC assessment (CNSC 2018) discusses the concept of half-life as it relates to radionuclides. Half-life is the length of time it takes for half of the radioactive atoms of a specific radionuclide to decay. Each radionuclide has its own unique half-life, which can range from milli-seconds to millions of years. Thus, depending on the radionuclide, a number of radionuclides would meet the definitions of “persistent” as outlined in the Persistence and Bioaccumulation Regulations under the CEPA. Moreover, research on stable and unstable (radioactive) isotopes of the same elements (e.g., Yankovich et al. 2013, Barnett et al. 2014) indicates that some radionuclides also meet the criteria necessary for classification as bioaccumulative.
2.2 Release: To what extent is the chemical substance released in the Great Lakes basin?
2.2.1 Are there releases?
As documented in the CNSC CMC assessment (CNSC 2018, section 3), there have been and continue to be a number of sources of radionuclide releases to the Great Lakes. Information is provided on natural background and nuclear weapons testing fallout in section 3.1, along with the results of studies and monitoring dating from the 1950s to the present. Industrial sources are presented, with specific attention placed on facilities regulated by the CNSC and information on their activities, specific radionuclides relevant to their activities, and environmental monitoring data in comparison to the relevant limits and or guidelines.
The CNSC CMC assessment (CNSC 2018) presented environmental data up to 2015. Facility-specific monitoring has continued since, and updated versions of the data tables (up to 2022) are provided in Appendix A. The CNSC has also taken additional measures to make release and environmental data more readily accessible to the public in recent years. This is presented further in 2.2.2 and 2.3.1.
The renomination makes specific mention of current waste storage along the Great Lakes shoreline as well as the potential for a long-term deep geological repository (DGR) to be located within the Great Lakes basin. Currently, all releases associated with waste storage are already monitored and accounted for in the monitoring data mentioned above. Any future DGR, should it receive approval, would be required to geologically isolate the waste from the Great Lakes—eliminating the negligible releases associated with current on-site waste storage and also removing the unlikely hazards of accidental releases from these above-ground storage facilities.
2.2.2 Are releases likely to increase in the future?
While the CNSC plays no role in either the development or promotion of nuclear technology, it must, as the primary regulator of nuclear activities, stay abreast of current and potential future activities. Canada is a mature nuclear nation (external, English only, PDF, 7.64 MB), active throughout the entire nuclear fuel cycle from mining and milling (Saskatchewan), fuel refining and fabrication (Ontario), and nuclear power generation (Ontario, New Brunswick), to nuclear science and technology research facilities (research reactors and/or particle accelerators in British Columbia, Saskatchewan and Ontario), including medical isotope research and development and the associated decommissioning and waste management. Canada has developed and operates its own unique reactor design (CANDU – Canada Deuterium Uranium) in use internationally and nationally, supplying approximately 15% of Canada’s total electrical energy. This national concentration of resources, materials, and expertise makes nuclear one of Canada’s most secure and independent supply chains.
Ontario is the second-largest producer of electricity in Canada, accounting for 24% of Canada’s generation in 2019 (CER 2023). Approximately 92% of electricity in Ontario was produced from zero-carbon sources, with nuclear dominating (59%), followed by hydroelectricity (24%), wind (8%), and solar (1%) Footnote 1 (CER 2023). To ensure the long-term stability of this zero-carbon energy mix, the Ontario government made a strategic decision in 2015 to pursue the refurbishment of 10 nuclear reactors, extending life spans for an additional 30+ years.
With the federal government’s Canadian Net-Zero Emissions Accountability Act (external) committing to net-zero greenhouse gas emissions by 2050, there has been a substantial increased interest in the role nuclear power generation can play in achieving this objective. Working towards net-zero greenhouse gas emissions will require significant electrification of industry and the economy, which will be self-defeating unless the energy source for the electricity is zero-carbon. The federal government, in recognition of future net-zero energy needs and the advanced maturity of Canadian nuclear science and technology, demonstrated their support for the use of advanced reactor designs such as those known as Small Modular Reactors (SMRs) to support the net-zero goal for the future (visit Canada’s Small Modular Reactor Action Plan (external)).
The field of medical isotope development and production can also be expected to increase in coming years. Of the approximately 200 medical isotopes in common use today, nearly all are artificially created using either nuclear research reactors or cyclotrons. Canada’s Chalk River Laboratories (CRL) National Research Experimental (NRX: 1947–1992) was the first reactor to demonstrate the feasibility of commercial production of medical isotopes. Canada’s National Research Universal Reactor (NRU: 1957–2018) was added to CRL and produced the medical isotopes Co-60, Re-99m, Xe-133, I-131, I-125, C-14, and Ir-192 Footnote 2. Despite the retirement of NRU in 2018, Canada continues to be a major developer and supplier of medical isotope production, for both national use and global export. Technological developments have also allowed the leveraging of the unique CANDU fueling design for medical isotope production. The Bruce nuclear reactors have been the major supplier of Co-60 to the world for the last 60 years and have recently received approval to prepare for production of lutetium-177, a new radiotherapy treatment for advanced prostate cancer.
Given the concentration of technologies and expertise in nuclear science and technology and the value they hold with respect to combatting climate change and maintaining and advancing the Canadian economy and the health system, it is reasonable to assume an increase in nuclear activities within the Great Lakes basin in the future. This does not necessarily mean actual physical releases to the Great Lakes will increase, as releases are dependent on the type of activity and can be expected to be significantly mitigated with advances in technology. Any future nuclear facilities that are considered designated projects (external) are subject to the Impact Assessment Act , under which any potential impacts would be assessed. Moreover, all proposed nuclear facilities undergo an environmental review and must supply an environmental risk assessment with their licence application to the CNSC in accordance with the Nuclear Safety and Control Act (NSCA) and associated regulations. If licenced, the facility will need to meet their relevant effluent, emissions, and environmental monitoring and reporting requirements.
It is indicated in the renomination proposal that the International Joint Commission (IJC) Nuclear Task Force concluded that “monitoring […] in the Great Lakes meets the need for compliance by users of radioactive materials with the conditions of the licenses” (IJC 1997). Since the publication of the IJC report, monitoring of both releases and the receiving environment has increased substantially, along with public accessibility of the results. This is in no small part due to the coming into force of the NSCA with its expanded environmental protection mandate, participant funding for the public hearing process, and requirements for licensee public engagement communications programs. Further information on these aspects of the NSCA were provided in the CNSC CMC assessment (CNSC 2018).
In this context, the statement in the renomination proposal that monitoring “does not provide a useful way for understanding the amounts of releases to the Great Lakes system” (page 5 of CELA and TFGLBN 2022) appears to be misinterpreting the findings of the IJC report. The IJC report did not conclude that there was a deficiency with respect to being able to estimate releases to the Great Lakes; rather, it proposed that little of the monitoring required at the time was “designed to address or are capable of considering the movement and cycling of radionuclides through environmental compartments and ecosystems” (IJC 1997). Since the findings of the IJC Nuclear Tasks Force report (IJC 1997), notable developments occurred in the environmental monitoring of radionuclides in the Great Lakes and elsewhere. The environmental protection mandate was expanded under the NSCA (section 5.2 and associated subsections in CNSC 2018), and there have been significant advances in international and national environmental radiation sciences through the last three decades and increased radiological monitoring and research in the Great Lakes.
Further clarification is also required regarding the statement in the renomination proposal that “a major part of this problem is that all facilities are not required to report on the same radionuclides” (page 5 of CELA and TFGLBN 2022). >Not all nuclear activities release the same suite of radionuclides, and therefore monitoring and reporting requirements differ among facilities; in fact, monitoring and reporting are site-specific in order to capture all relevant radionuclides and ensure adequate protection of human health and the environment surrounding the site. As an example, even nuclear power plants of differing basic designs (e.g., U.S.-based light-water reactors versus Canadian-based heavy water reactors) have slightly different radionuclide release profiles. Section 3 of the CNSC CMC assessment (CNSC 2018) provides a breakdown of each of the major industrial sources of radionuclide releases to the Great Lakes, with a description of the specific types of radionuclides each activity is associated with and thus responsible for monitoring.
Comprehensive monitoring and reporting requirements have resulted in large quantities of data on releases of both hazardous and nuclear substances in the Great Lakes basin. This information is reported on annually in Regulatory Oversight Reports (RORs) through the public Commission meeting process and the CNSC’s Environmental Protection Review Reports and in publicly available downloadable radionuclide release databases (external). The latter two are available both through the CNSC website and on the federal Open Government Portal. Provincial programs include the Government of Ontario’s Ontario Reactor Surveillance Program (ORSP) (external), which has fixed monitoring stations located around the nuclear facilities in Ontario and near Fermi II Nuclear Generating Station. The ORSP routinely samples air, drinking water, and foodstuff (including milk) to be analyzed for radionuclides. The program produces annual reports; however, these are not currently available on the website. In addition, Ontario’s Drinking Water Surveillance Program (DWSP) (external) monitors water quality in selected municipal drinking water systems for scientific and research purposes, including radiological parameters such as gross alpha and beta and tritium.
Publicly available data clearly indicates that releases to the Great Lakes are extremely well quantified across multiple nuclear subsectors. Hence, the statement of “it is currently impossible to state the extent of the releases of radionuclides into the Great Lakes basin” (page 6 of CELA and TFGLBN 2022) is incorrect.
2.3 Levels: Are levels of the chemical substance harmful, or likely to become harmful, in the Great Lakes environment?
2.3.1 Are measured levels meeting or exceeding benchmarks or guidelines for the protection of wildlife and humans, including fish consumption advisory levels, water quality standards, etc.?
The CNSC CMC assessment (CSNC 2018, section 2.3) provided a summary of radiation health effects along with the internationally and nationally recognized limits, benchmarks, and guidelines associated with protection of the public and the environment (including non-human biota). The previous report also documents the means by which this international guidance is revised and updated as science and technology evolve. This information remains valid.
Clarification is warranted regarding a statement in the renomination report that “the benchmarks just referred to are based on the assumption that there is an acceptable level of exposure” (page 7 of CELA and TFGLBN 2022). The international and national regulatory dose limits are based on the application of the Linear-No-Threshold (LNT) model and thus, in contrast to the statement, do not assume an acceptable level of exposure. For further discussion on the various dose-response models and the role of the LNT, the reader is referred to the CNSC CMC assessment (CNSC 2018, section 2.3.1, Fig. 4), where this information is presented in detail.
No licensed nuclear facilities associated with the Great Lakes basin have exceeded licence release limits or the regulatory public dose limit, as documented in the CNSC CMC assessment (CNSC 2018) and in CNSC Annual Regulatory Oversight Reports, which are publicly available. Environmental monitoring data of surface waters, associated drinking water plants, and groundwater demonstrate that radionuclide levels are well below applicable drinking water standards and guidelines.
The results of the CNSC Independent Environmental Monitoring Program (CNSC 2018, section 5.2.2) similarly demonstrate protection of human health and the environment and are available on the CNSC website, accessible on the federal Open Government Portal (external), and searchable on the Natural Resource Canada Open Science and Data Platform (external).
The licensees’ effluent and receiving environment monitoring programs, along with their site-specific environmental risk assessments (ERAs) required under their CNSC licence, demonstrate that the environment associated with these facilities is protected. This includes sampling and analysis of abiotic (e.g., air, water, soil) and biotic (e.g., crops, milk, fish) media for radionuclides to estimate dose to both members of the public and non-human biota (e.g., plants, fish, and wildlife) that may come into contact with or consume these media.
Public doses reported for Canadian nuclear facilities (CNSC 2018, sections 3.2.4 and 4.1; Annual RORs; radionuclide release database) are at least an order of magnitude lower than the public regulatory dose limit of 1 mSv/year. This is in part due to the CNSC requiring licensed nuclear facilities to not only meet the dose limit but also keep radiological doses to members of the public as low as reasonably achievable (ALARA), in line with a precautionary approach. Licensees are required to demonstrate that best engineering and operating principles are applied in accordance with the principle of ALARA. Therefore, the statement within the renomination proposal that “NRC bases protection standards on engineering principles primarily, not public health […] The situation is similar in Canada” (page 7 of CELA and TFGLBN 2022) is inaccurate, as principles of engineering and public health are not mutually exclusive in the pursuit of ALARA.
With the coming into force of the NSCA in 2000, the CNSC became the first nuclear regulatory body in the world to require demonstration not only of protection of the public but also of the environment, including non-human biota. This principle has since been adopted by the International Commission on Radiological Protection (ICRP), the International Atomic Energy Agency (IAEA), and many national nuclear regulatory authorities, resulting in a wealth of international and national guidance and research on environmental radiation protection (CNSC 2018, section 2.3.3).
Nuclear licensees in Canada must be compliant with the Canadian Standards Association (CSA) Environmental Risk Assessment standard (N288.6:22), in which clause 7.4.2, on radiological benchmarks, states that benchmarks for quantitative effects assessment should follow UNSCEAR (2008) (i.e., 100 µGy/h [2.4 mGy/d] for terrestrial biota and 400 µGy/h [9.6 mGy/d] for aquatic biota). The UNSCEAR (2008) report states that chronic exposures to low-linear energy transfer radiation at dose rates of less than 100 µGy/h (2.4 mGy/d) to the most highly exposed individuals would be unlikely to have significant effects on most terrestrial animal populations and that maximum dose rates of 400 µGy/h (9.6 mGy/d) to a small proportion of the individuals in aquatic populations of organisms would not have any detrimental effects at the population level. Total dose rate from the sum of radionuclides measured in the receiving environment is also compared to these benchmarks, which is a more conservative approach than the benchmark per chemical approach, which is used for other contaminants of concern.
This advancement with the application of radiation benchmarks in Canadian nuclear standards reinforces that the call for a “revised monitoring and analytical protocol with emphasis on bio-uptake characteristics, physiological roles and impacts” of the IJC Nuclear Task Force (IJC 1997) was adequately addressed.
2.3.2 Are concentrations in the Great Lakes environment increasing, suggesting early action is warranted?
The history of radionuclide monitoring in the Great Lakes basin, as outlined and discussed in the CNSC CMC assessment (CNSC 2018, section 3.0), demonstrates that most anthropogenic radionuclide levels in the Great Lakes have decreased relative to the peaks in the 1960s because weapons fallout radionuclides have decayed.
Of specific interest is the historical and current monitoring record for tritium. Tritium is produced both naturally in the atmosphere and from anthropogenic sources, including weapons testing and nuclear power plant operation (especially the Canadian CANDU reactors). Through compilation of data from several sources between 1953 and 2008, the CNSC CMC assessment (CNSC 2018, section 3.1) demonstrated that the maximum concentrations of tritium in all of the Great Lakes peaked prior to the start of operation of Canada’s nuclear power plants. Since this peak in the 1960s, tritium concentrations continued to decrease despite the operation of nuclear power plants. This conclusion is further supported by two recent scientific publications investigating tritium levels in the Great Lakes (King-Sharp and Frape 2019; Dove et al. 2021). The most recent Great Lakes-wide tritium survey was completed in 2017–2019 in partnership with ECCC’s Great Lakes water quality monitoring cruises (Dove et al. 2021). This survey confirmed past findings, documenting a 20-year trend of declining tritium levels with a “marked overall reduction in tritium values since maxima in the late 1960s.”
Further evidence of decreased radionuclide emissions associated with nuclear power plants is demonstrated in recent research measuring carbon-14 (C-14) in tree rings (Vogel et al. 2022). Annual tree ring C-14 composition was strongly correlated with reported emissions from associated nuclear power plants (Vogel et al. 2022). These findings served to verify the reliability of emissions reporting and allowed estimation of emissions back through to the 1990s, when C-14 emission data were not available for the nuclear power plants. In this way, Vogel et al. (2022) demonstrated that current emissions were three to four times lower compared to the 1990s.
2.4 Route of exposure: Are the Great Lakes a route of exposure to humans or wildlife for this chemical substance, and are impacts or likely impacts caused by routes of exposure?
2.4.1 Water, sediments, and/or air deposition?
From a public health perspective, radiation exposures to the public from environmental releases of radionuclides from Canadian nuclear power plants represent a small fraction of natural background radiation (Lane et al. 2013). Radiation dose to members of the public is approximately equally distributed between natural background radiation and anthropogenic radiation; however, the anthropogenic dose is overwhelmingly a result of medical usage (48%) as opposed to industrial exposure (0.1%) (NCRP 2009).
From an environmental perspective, releases to the Great Lakes from licensed nuclear facilities are an exposure pathway for local aquatic biota. Therefore, the CNSC requires licensees to complete and maintain environmental risk assessments (ERAs) informed by site-specific monitoring of releases and radionuclide levels in the receiving environment. The comprehensive ERAs consider multiple abiotic (e.g., air, water, sediment) and biotic (e.g., food, wildlife) environmental compartments (Figure 1) and incorporate transfer factors between compartments, including for bioaccumulation, for both humans and non-human biota. Multiple exposure pathways are evaluated, as the primary pathway varies depending on the specific radioisotope and human or ecological receptor (e.g., public, wildlife, benthic invertebrates, fish). The environmental monitoring programs and ERAs for Canadian licensed nuclear facilities around the Great Lakes indicate that there are no measurable negative effects on the aquatic environment from radiological exposures.
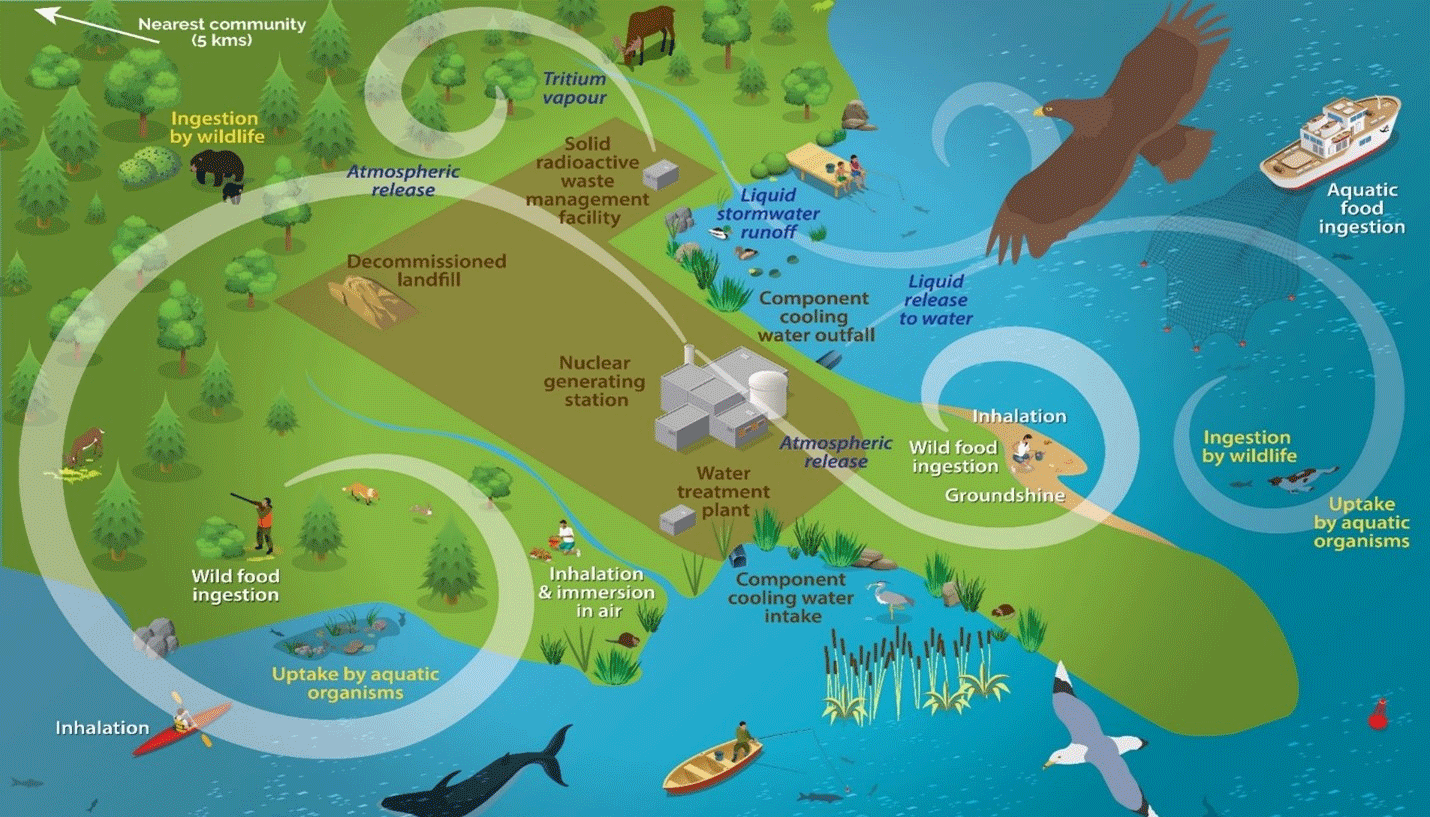
Description
The figure shows a conceptual model used in the environmental risk assessment of a generic nuclear power generating station site. The environmental risk assessment identifies, quantifies, and characterizes the risk posed by contaminants (nuclear or hazardous substances) and physical stressors in the environment. The conceptual model is used to succinctly describe the relationship between contaminants/physical stressors and receptors (humans and biota), emphasizing the pathways through which exposure can occur. In this context, the figure shows an example generic nuclear power station located near a forest and on the shore of a large waterbody. The station has supporting infrastructure including a water treatment plant, solid radioactive waste management facility, and decommissioned landfill. The figure illustrates the station’s releases and interactions with the environment. For example, atmospheric releases, such as tritium vapour, can be inhaled by humans and animals located in the vicinity of the station. Several potential receptors are considered, including a person gathering plants, a person hunting wildlife, a person kayaking, a person fishing, and a person playing at a beach. Moreover, liquid releases to water through the station’s cooling water outfall and liquid stormwater runoff from the station property can be taken up or ingested by aquatic organisms. The risk assessment also considers exposure through the food chain, including ingestion of wild foods by humans.
Figure 1 Example conceptual model used in the ERA of a generic nuclear generating station site.
2.5 Scale: Does the geographic scale of the levels of the chemical substance in the Great Lakes have binational significance?
2.5.1 Is the contamination currently, or likely to become, lakewide or multi-lake in scale as opposed to localized?
The suite of current radionuclide release and receiving environment monitoring programs demonstrate that there are, for some radionuclides (e.g., tritium), some facilities (e.g., nuclear power plants) producing measurable localized increases in lake activity levels, all of which are below levels of concern. Despite decades of operation of nuclear facilities along the shores of the Great Lakes, the only evidence of lake-wide distribution of radionuclides is associated with historical nuclear weapons testing. Under the current extensive regulatory regime and the advances in nuclear waste management treatment and control technology, the approval of any facility with the potential for lake-wide contamination is not envisioned.
2.5.2 Does the contamination have the potential to cause binational transboundary impacts?
Southern Ontario supports and benefits from a diverse and complex range of nuclear activities, as documented in the CNSC CMC assessment (CNSC 2018). There is also the possibility of further expansion of the nuclear sector in this region to meet current and future electricity needs and climate change objectives, as well as to ensure access to advanced nuclear medicine (section 2.2.2). However, current and potential future activities do not support a conclusion of lake-wide or binational transboundary impacts. Research and regulatory monitoring and risk assessment activities demonstrate that radionuclide footprints associated with Canadian nuclear facilities in the Great Lakes are localized and that both the environment and the public are protected (CNSC 2018). The current Canadian nuclear regulatory regime is specifically designed to ensure this is the case now and continues to be in the future (CNSC 2018; section 5.2).
2.6 Management: To what extent are the uses and releases of the chemical substance controlled/managed?
2.6.1 Are programs and management actions for the chemical substance currently in place at the local, state/provincial, tribal, Indigenous, federal or international level?
As recognized by the renomination proposal (CELA and TFGLBN 2022) and clearly demonstrated in the CNSC CMC assessment (CNSC 2018, section 5.0), there is a comprehensive international and national regulatory framework with respect to the safe regulation of nuclear activities. The CNSC establishes a comprehensive network of international and national arrangements and agreements with nuclear, emergency planning, public health, and environmental agencies and organizations. The formal agreements and/or arrangements are documented and made available to the public on the CNSC website (international cooperation and domestic arrangements). This is especially true for emergency planning, public health, and environment, as these issues not only cross organizational mandates within the federal government but also overlap with federal and provincial responsibilities. The depth and complexity of the working relationships between the CNSC and other federal and provincial agencies (refer to CNSC 2018, section 5.3) is inadequately represented in the statement in the renomination proposal that “the environmental and health agencies in both countries take a back seat to the CNSC” (page 10 of CELA and TFGLBN 2022).
Health Canada, specifically the Environmental and Radiation Health Sciences Directorate (external), brings together environmental health and radiation regulation, health research and surveillance, chemical surveillance and monitoring, and science policy. Residing within this Directorate is the Radiation Protection Bureau (RPB) (external), which serves as Canada’s national centre of excellence for environmental radiation protection and occupational radiation dosimetry, manages radiation surveillance and monitoring networks (e.g., Canadian Radiation Monitoring Network, refer to CNSC 2018, section 5.3.3), and conducts research on exposure trends and health outcomes of occupational exposures to radiation. The RPB is also responsible for the National Dosimetry Service and National Dose Registry—the single largest, most comprehensive national database of occupational exposure data available globally. Lastly, the RPB coordinates Canada’s preparedness for nuclear emergencies as the lead agency for the Federal Nuclear Emergency Plan.
The CNSC and Health Canada have an extensive working relationship, which has been formally documented in a Memorandum of Understanding (MOU). Of most direct relevance to the relationship is the coordination with respect to the Canadian Radiological Monitoring Network (CRMN) (external) and cooperation and participation with respect to radiological health and epidemiological research, both internationally and nationally. The CRMN was discussed in the CNSC CMC assessment (CNSC 2018, section 5.3.3). Therefore, the only point that will be re-emphasized here relates to the concentration of Health Canada monitoring stations associated with each of Canada’s nuclear power plants. The MOU specifically addresses the commitments of both agencies to share information related to the Health Canada CRMN and the CNSC’s Independent Environmental Monitoring Program (IEMP) and licensee programs. The analytical sensitivity of the Health Canada CRMN is especially important as it provides an independent (i.e., of both licensee and CNSC) means of rapid detection of deviations from normal releases from Canada’s nuclear power plants and can be used to cross-verify licensee emissions reporting.
Health Canada plays a key role in radiation health and epidemiological research through their internal research programs, their participation in international radiation science organizations such as the United Nations Scientific Committee on the Effects of Atomic Radiation (UNSCEAR) (external, English only) and ICRP, and joint research initiatives either directly with the CNSC or indirectly through each organization’s role and participation in the Federal Nuclear Science and Technology (FNST) program (external).
The CNSC Directorate of Environmental and Radiation Protection and Assessment retains a team of health science professionals consisting of radiation biologists and epidemiologists whose role is to ensure the CNSC regulatory framework and licensing decisions are informed by the latest developments in radiation and health science. CNSC health science specialists maintain credibility and expertise in these fields through solid cross-communication between themselves and Health Canada scientists, and through active joint participation in international organizations such as UNSCEAR and ICRP, as well as involvement in and publishing of radiation health research. An example of the latter is the Canadian Organization on Health Effects from Radiation Exposure (COHERE).
COHERE was established as a joint initiative between Health Canada and the CNSC to improve understanding of the potential health impacts from exposure to low doses of radiation. It was formed with the following objectives:
- Better align Health Canada’s and the CNSC’s radiation science research priorities to focus and leverage resources
- Maintain and enhance expertise in radiobiology and epidemiology within the Government of Canada
- Provide an informed and consistent federal message to the public and stakeholders on matters involving low dose/dose-rate ionizing radiation
- Strengthen Canada’s contribution towards international efforts on the assessment of ionizing radiation doses and effects for application in international recommendations, Canadian regulations and national guidance
Another example of cross-departmental collaboration is the Federal Nuclear Science and Technology program (external) (CNSC 2018, section 5.3.5). The research advisory Committee for “Theme 1: Supporting the development of biological applications and understanding the implications of radiation on living things” is co-chaired by Health Canada, and the CNSC health science, dosimetry and radiation protection specialists participate as Committee members.
Similarly, the working relationship between ECCC and CNSC environmental protection specialists and regulatory scientists is well developed and managed through their MOU, which is publicly available on the CNSC website. The CNSC and ECCC work closely to ensure that both of their respective mandates are achieved and respected. At the applied levels, this involves direct cooperation on matters of mutual concern such as ensuring that environmental protection under the NSCA respects federal core environmental legislation such as the CEPA, Fisheries Act (FA), Species at Risk Act (SARA), and Migratory Birds Convention Act (MBCA).
Working protocols have been established between the CNSC and ECCC to assist both parties where shared responsibilities and regulations merge, such as for reviews, programs, and regulatory compliance verification. This cooperation involves providing information to support each other’s compliance and/or enforcement action, participating in joint inspections, and establishing protocols to ensure the reporting of spills and/or environmental emergencies is made to both agencies. An annual workshop is coordinated between the parties to exchange knowledge on current and forthcoming legislation and relevant environmental science and to support the joint development of annual work plans. ECCC is a regularly attendee at CNSC Commission hearings to provide the independent Commission members with the opportunity to directly query ECCC representatives on issues of parallel or shared responsibilities when making licencing decisions. Additionally, CNSC and ECCC both serve on the advisory committee directing nuclear-related environmental research as part of FNST “Theme 2: Supporting environmental stewardship and radioactive waste management”.
While MOUs commit to a spirit of cooperation, they in no way limit or impair the responsibility of each party for meeting their respective mandates. This present document serves as example of this cooperation, with the CNSC assisting ECCC through the provision of their technical expertise and advice. However, the decision with respect to the CMC classification is the responsibility of ECCC upon weighing all evidence.
2.6.2 Are current actions adequate, and/or do gaps exist?
The first point in the renomination proposal (CELA and TFGLBN 2022, page 10) criticizes the lack of application of the guiding principles of the GLWQA, specifically referring to concepts of “persistent toxic substances,” “precautionary approach,” “prevention,” “zero discharge,” and “virtual elimination.” A response to each principle is provided below.
An inherent characteristic of all radionuclides is that, after some radionuclide-specific period of time, they become stable and therefore non-radioactive. A number of radionuclides have half-lives long enough to be classified as “persistent.” This concept was discussed in the CNSC CMC assessment (CNSC 2018; section 2.1) and reiterated earlier in section 2.1.2. It is important to note that, while a number of radionuclides would be classified as persistent, they have not been classified as persistent toxic substances as defined under the CEPA, a process that implicitly applies the above-mentioned concepts.
The core principles within the CEPA are specifically recognized within the CNSC environmental protection framework, as documented in REGDOC 2.9.1 (CNSC 2020) and as presented in the CNSC CMC assessment (CNSC 2018, section 5.2.1):
“The environmental protection program consists of an environmental policy with commitments to the application of ALARA (for radionuclides) and best available technology economically achievable (BATEA) (for hazardous substances), the “polluter pays” and precautionary principles, and the concepts of pollution prevention, sustainable development and adaptive management.”
Thus, the principles of the precautionary approach and prevention are recognized and applied in practice by the CNSC.
The risk management measures referred to as zero discharge and virtual elimination are not applicable at this point of the decision-making process. As clearly stated in the GLWQA (2012), these risk management concepts apply to substances that have been confirmed as being CMCs, and only where considered “appropriate.”
Regarding the second point in the renomination proposal (CELA and TFGLBN 2022, page 10), which questions the use of ALARA, the reader is referred to explanations in section 2.3.1.
The third point in the renomination proposal (CELA and TFGLBN 2022, page 10) states a lack of substantial involvement of the respective country’s environmental and health agencies in the decision-making by the CNSC. This point has been addressed in section 2.6.1.
With respect to the adequacy of monitoring and reporting mentioned in the fifth point (CELA and TFGLBN 2022, page 10), it should be clarified that, since the referenced IJC Nuclear Tasks Force report (IJC 1997), substantial advancements occurred over the last 20+ years in both licensee and governmental monitoring, reporting, and research activities. Since the coming into force of the NSCA, with its enhanced environmental protection mandate, both ERA requirements and associated effluent and receiving environment monitoring have been required of licensees (CNSC 2018, section 5.2.1). Additional monitoring is performed through the CNSC’s IEMP (CNSC 2018, section 5.2.2), the provincial Ontario Reactor Surveillance Program (CSNC 2018, section 5.3.2), and the Health Canada CRMN (CNSC 2018, section 5.3.3). It was recognized in the CNSC CMC assessment (CNSC 2018, Table 13) that all stakeholders would benefit from an increased awareness of and access to the results of these activities. Since then, substantial improvements are evident in public access to information, as outlined in sections 2.2.2 and 2.3.1.
The sixth point claims that “the CNSC, by its own admission, would hold nuclear operating licence applicants to a lower standard than the Ontario Ministry of Environment and Climate Change does ECA [Environmental Compliance Approval] applicants” (CELA and TFGLBN 2022, page 11). A review of the referenced source document for this statement (Blaise and Khan 2022) indicates that the authors have inappropriately compared two completely different safety elements and regulatory practices. The source document (Blaise and Khan 2022) in this context speaks to the Safety and Control Area (SCA) of “Fitness for Service” (covering activities that affect the physical condition of structures, systems, and components to ensure that they remain effective over time) instead of “Environmental Protection”; thus, the comparison to the Ontario Environmental Compliance Approval process is not applicable. Furthermore, the facility in question was in compliance with all their radiation and environmental protection requirements. In fact, this is a demonstration of the in-depth and public regulatory process employed by the CNSC compared to other regulatory bodies. The CNSC regulatory framework extends well beyond the limited aspects associated with Ontario Environmental Compliance Approvals—addressing and publicly reporting to the Commission on fourteen technical topics referred to as SCAs.
The statement with regard to “blurred roles and improperly conflated responsibilities for safety regulation versus nuclear technology promotion” (CELA and TFGLBN 2022, page 11) appears to be a misrepresentation of the mandate of the NSCA and the responsibilities of the CNSC. In fact, a core strength of the Canadian nuclear regulatory system, which has been recognized internationally (IAEA 2019), is the independence of the CNSC. The NSCA and the CNSC are structured and operate such that they are independent of political influence and play no role in the promotion or development of nuclear technology (CNSC 2018, section 5.2). The structure not only ensures external independence but also provides independent decision-making between the Commission members themselves and CNSC staff as elaborated in the following.
The Commission consists of two distinct entities:
- the Commission, which refers to the organization’s tribunal component, and
- the CNSC, which refers to the corporate body and the staff in general.
The Commission is an independent administrative tribunal and court of record. Commission Members are appointed by the Governor in Council of Canada for terms not exceeding five years and may be re-appointed. Appointed Members are independent of all influences, including political, governmental, special interest, or private sector. The Commission is the “decision-making body” with respect to regulatory policy, regulations, and licensing decisions of nuclear-related activities in Canada.
CNSC staff provide the administrative management and organizational functions necessary to fulfill the mandate of the NSCA. This includes, but is not limited to, the preparation of proposals related to policy and regulation along with recommendations on licensing decisions, the presentation of these to the Commission for consideration during public proceedings, and the subsequent administration of the Commission’s decisions (e.g., licensing certification, compliance inspections, enforcement action).
The President, who also serves as the Chief Executive Officer of the CNSC, is the only Commission Member to have a direct working relationship with CNSC staff. Communications between CNSC staff and the remaining Commission members are restricted to official public Commission hearings and meetings. In this manner, the Commission rules on a decision based on the information heard and made available through this public process, including the information submitted by proponents (e.g., licence applicants) and intervenors (e.g., members of the public, non-governmental and civil society organizations, Indigenous representatives).
Thus, the CNSC, both Commission and staff, play no role in energy planning or in the development or promotion of nuclear technologies. The sole mandate of the CNSC is the regulation of nuclear activities with respect to safety, and the protection of human health and the environment, and Canada’s associated international obligations.
The last aspect in this section of the renomination that merits direct response relates to independent technical research capacity and the claim that “the regulator is beholden to industry and their contractors for its state of knowledge” (CELA and TFGLBN 2022, page 11). In clear contrast to this claim, the CNSC has extensive and multiple mechanisms for ensuring their assessments and recommendations are based on solid scientific principles and research. This includes, but is not limited to, the following:
- Internal in-house scientific and engineering expertise provided by the CNSC Technical Support Branch
- Internally funded and directed research managed and/or completed by CNSC scientists and engineers
- Authority under the NSCA to establish independent scientific and technical advisory bodies, such as:
- Obligation to consult with experts from different federal authorities on subjects under their mandates (e.g., Memoranda of Understanding with Health Canada and Environment and Climate Change Canada). Thus, CNSC staff recommendations are often made with considerable input/expertise from multiple federal authorities.
- CNSC specialist staff support and participation in international (e.g., UNSCEAR, ICRP, IAEA) and national (e.g., COHERE) scientific and regulatory organizations
- Coordination and participation in external international and national research entities such as the FNST and targeted research directed through the Canadian Natural Sciences and Engineering Research Council of Canada (NSERC) ALLIANCE program (e.g., Small Modular Reactors Research Grant Initiative (external))
The full breadth of these activities is too involved to address in detail here. Therefore, a few key examples are presented below.
An excellent example of CNSC activities to ensure CNSC scientific credibility and independence is comprehensively documented on the CNSC research on geological repositories website. This is an example of the CNSC’s long-term planning to ensure that CNSC staff have the technical expertise and access to the underlying science to independently assess any future proposal for a deep geological repository in Canada.
Another example would be the CNSC’s scientific and technical focus on tritium management and environmental and health studies. Because of the importance of tritium in the Canadian nuclear sector, the CNSC maintains a dedicated tritium website. This includes the suite of reports and scientific publications associated with the tritium studies project launched in 2007 at the request of the Commission, as well as direct links to the latest tritium scientific publications and research completed in Canada or by Canadian researchers since 2011 to the present (38+ publications, including the latest research on tritium in the Great Lakes). This work has involved the CNSC internal research and support program, direct research by CNSC science and technical staff, coordination with Health Canada researchers, and directed research through the FNST.
The results of these research activities and the scientific and technical credibility of CNSC staff can be demonstrated through a review of the published peer-reviewed scientific literature. A SCOPUS Footnote 3 review identifies over 488 individual publications since 2000, with 141 publications over the last five years (2018–2022), as being authored/coauthored by CNSC staff.
3. Renomination comments on the 2018 Canadian Nuclear Safety Commission chemicals of mutual concern assessment
The renomination report (CELA and TFGLBN 2022, page 11) expresses discomfort with the CNSC ruling on whether radionuclides are considered for classification as CMCs under the binational agreement. As previously stated, established working relationships and formal MOUs in no way replace each agency’s needs to meet their own independent mandates. The CNSC, as part of the working relationship with ECCC, has been asked to provide advice and recommendations on this issue. The decision as to considering radionuclides as CMCs will be made by ECCC and their binational partners and not the CNSC.
4. Conclusions and recommendations
The very nature of risk assessment is to recognize and prioritize risks such that limited financial and scientific resources are focussed on priority issues. Key to the decision as to whether radionuclides should be considered CMCs is whether radionuclides merit even further governmental and scientific oversight than is currently in place.
Based on a review of the renomination report, the CNSC’s conclusions remain as provided in its previous CMC assessment (CNSC 2018).
“When the comprehensive nature of the current regulatory framework and national monitoring and research network is considered, it is clear that activities are already in place federally which would satisfy all Annex 3 commitments for designated CMCs. Therefore, based on the evaluation summarized in this report, the CNSC has concluded that:
- the health and safety of persons and the environment associated with the Great Lakes are protected
- designating radionuclides as a CMC would not lead to or enable any additional action on the part of the Canadian federal government
As such, it is recommended that Canada does not support the nomination of radionuclides as a candidate CMC for further evaluation.”
5. References
Barnett CL, Beresford NA, Walker LA, Baxter M, Wells C, Copplestone D. 2014. Transfer parameters for ICRP reference animals and plants collected from a forest ecosystem. Radiation and Environmental Biophysics. Vol. 53: 125–149.
Blaise K, Khan R. 2022. Designating Radionuclides as a Chemical of Mutual Concern under the Canada-United States Great Lakes Water Quality Agreement: Moving Towards Greater Oversight, Environmental Protection and Transparent, Open-sourced Science. 16 pages.
CELA (Canadian Environmental Law Association) and TFGLBN (Toxics-Free Great Lakes Binational Network). 2022. Renomination of radionuclides as chemicals of mutual concern under the Great Lakes Water Quality Agreement. Prepared by John Jackson. 18 pages.
CELA (Canadian Environmental Law Association). 2016. Radionuclides as a chemical of mutual concern in the Great Lakes basin. Prepared by John Jackson. 20 pages.
CER (Canada Energy Regulator). 2023. Provincial and territorial energy profiles – Ontario (Website). Accessed 26 June 2023. Last updated 09 June 2023.
CNSC (Canadian Nuclear Safety Commission). 2020. REGDOC-2.9.1: Environmental Protection: Environmental Principles, Assessments and Protection Measures. Version 1.2.
CNSC (Canadian Nuclear Safety Commission). 2018. Assessment of the Relevance of the Inclusion of Radionuclides as a Chemical of Mutual Concern under Annex 3 of the Canada-United States Great Lakes Water Quality Agreement. Canadian Nuclear Safety Commission, Ottawa, Canada. ISBN 978-0-660-24584-3.
CNSC (Canadian Nuclear Safety Commission) and EC (Environment Canada). 2009. Risk management of uranium releases from uranium mines and mills 2007 annual report. Canadian Nuclear Safety Commission INFO-0771. Ministry of Public Works ISBN 978-1-100-13751-1.
Dove A, Backus SM, King-Sharp K. 2021. Tritium in Laurentian Great Lakes surface waters. Journal of Great Lakes Research. Vol. 47(5): 1458–1463.
ECCC (Environment and Climate Change Canada) and HC (Health Canada). 2006. Priority Substances List Assessment Report. Releases of radionuclides from nuclear facilities (Impact on Non-human Biota).
Gillies M, Richardson DB, Cardis E, Daniels RD, O’Hagan JA, Haylock R, Laurier D, Leuraud K, Moissonnier M, Schubauer-Berigan MK, Thierry-Chef I, Kesminiene A. 2017. Mortality from Circulatory Diseases and other Non-Cancer Outcomes among Nuclear Workers in France, the United Kingdom and the United States (INWORKS). Radiation Research. Vol. 188(3): 276–290.
GLWQA (Great Lakes Water Quality Agreement). 2012. Protocol Amending the Agreement Between Canada and the United States of America on Great Lakes Water Quality, 1978, as Amended on October 16, 1983, and on November 18, 1987. 56 pages.
Hamra GB, Richardson DB, Cardis E, Daniels RD, Gillies M, O’Hagan JA, Haylock R, Laurier D, Leuraud K, Moissonnier M, Schubauer-Berigan M, Thierry-Chef I, Kesminiene A. 2016. Cohort profile: The International Nuclear Workers Study (INWORKS). International Journal of Epidemiology. Vol. 45(3): 693–699.
IAEA (International Atomic Energy Agency). 2019. Report of the Integrated Regulatory Review Service (IRRS) mission to Canada. IAEA-NS-IRRS-2019/05. 117 pages.
IJC (International Joint Commission). 1997. Inventory of Radionuclides for the Great Lakes. IJC Nuclear Task Force. 121 pages.
King-Sharp KJ, Frape SK. 2019. Fate and transport of tritium in the Laurentian Great Lakes system. Journal of Great Lakes Research. Vol. 46 (3): 500–507.
Lane R, Dagher E, Burtt J, Thompson PA. 2013. Radiation Exposure and Cancer Incidence (1990 to 2008) around Nuclear Power Plants in Ontario, Canada. Journal of Environmental Protection. Vol. 4(9): 888–913.
Laurier D, Richardson DB, Cardis E, Daniels RD, Gillies M, O’Hagan J, Hamra GB, Haylock R, Leuraud K, Moissonnier M, Schubauer-Berigan MK, Thierry-Chef I, Kesminiene A. 2017. The International Nuclear Workers Study (INWORKS): A Collaborative Epidemiological Study to Improve Knowledge About Health Effects of Protracted Low-Dose Exposure. Radiation Protection Dosimetry. Vol. 173(1): 21–25.
Leblanc JE, Burtt, JJ. 2019. Radiation Biology and its Role in the Canadian Radiation Protection Framework. Health Physics . Vol. 117(3): 319–329.
Leuraud K, Richardson DB, Cardis E, Daniels RD, Gillies M, O’Hagan JA, Hamra GB, Haylock R, Laurier D, Moissonnier M, Schubauer-Berigan MK, Thierry-Chef I, Kesminiene A. 2015. Ionising radiation and risk of death from leukaemia and lymphoma in radiation-monitored workers (INWORKS): An international cohort study. The Lancet Haematology. Vol. 2(7): e276–e281.
Leuraud K, Richardson DB, Cardis E, Daniels RD, Gillies M, Haylock R, Moissonnier M, Schubauer-Berigan MK, Thierry-Chef I, Kesminiene A, Laurier D. 2021. Risk of cancer associated with low-dose radiation exposure: comparison of results between the INWORKS nuclear workers study and the A-bomb survivors study. Radiation and Environmental Biophysics. Vol. 60(1): 23–39.
NCRP (National Council on Radiation Protection and Measurements). 2009. Report No. 160, Ionizing Radiation Exposure of the Population of the United States. ISBN 978-0-929600-98-7.
Richardson DB, Cardis E, Daniels RD, Gillies M, O’Hagan JA, Hamra GB, Haylock R, Laurier D, Leuraud K, Moissonnier M, Schubauer-Berigan MK, Thierry-Chef I, Kesminiene A. 2015. Risk of cancer from occupational exposure to ionising radiation: retrospective cohort study of workers in France, the United Kingdom, and the United States (INWORKS). BMJ. 351:h5359.
Richardson DB, Cardis E, Daniels RD, Gillies M, Haylock R, Leuraud K, Laurier D, Moissonnier M, Schubauer-Berigan MK, Thierry-Chef I, Kesminiene A. 2018. Site-specific Solid Cancer Mortality After Exposure to Ionizing Radiation: A Cohort Study of Workers (INWORKS). Epidemiology. Vol. 29(1): 31–40.
Thierry-Chef I, Richardson DB, Daniels RD, Gillies M, Hamra GB, Haylock R, Kesminiene A, Laurier D, Leuraud K, Moissonnier M, O’Hagan J, Schubauer-Berigan MK, Cardis E. 2015. Dose Estimation for a Study of Nuclear Workers in France, the United Kingdom and the United States of America: Methods for the International Nuclear Workers Study (INWORKS). Radiation Research. Vol. 183(6): 632–642.
Vogel F, Crann C, Pisaric MFJ. 2022. Tracking local radiocarbon releases from nuclear power plants in southern Ontario (Canada) using annually-dated tree-ring records. Anthropocene. Vol. 38: 100338.
Yankovich T, Beresford NA, Fesenko S, Fesenko J, Phaneuf M, Dagher E., Outola I, Andersson P, Thiessen K, Ryan J, Wood MD, Bollhöfer A, Barnett CL, Copplestone D. 2013. Establishing a database of radionuclide transfer parameters for freshwater wildlife. Journal of Environmental Radioactivity. Vol. 126: 299–313.
Appendix A. Updated data tables and figures
The following data tables and figures provide updated data as available, as compared to the CNSC CMC assessment (CNSC 2018). Table and Figure numbers are aligned with CNSC 2018.
Table 3: Annual average and maximum uranium and radium-226 values for Blind River Refinery Lake Huron water samples from 2006 to 2022. The method detection limit (MDL) is reported for values below the MDL. For comparison, uranium guideline values are 20 µg/L in drinking water1 and 15 µg/L for the protection of aquatic life.2 The radium-226 guideline value is 0.5 Bq/L in drinking water.3
Sampling year | Uranium annual average (µg/L) | Uranium annual maximum (µg/L) | Radium-226 annual average (Bq/L) | Radium-226 annual maximum (Bq/L) |
---|---|---|---|---|
2006 | 0.22 | 0.31 | <0.0005 | 0.0006 |
2007 | 0.2 | 0.28 | <0.0005 | <0.0005 |
2008 | 0.51 | <0.9 | 0.0006 | 0.0008 |
2009 | 0.21 | 0.21 | <0.0005 | <0.0005 |
2010 | 3.1 | 8.9 | <0.0008 | 0.002 |
2011 | 0.4 | 0.5 | 0.0006 | 0.0007 |
2012 | 0.2 | 0.3 | <0.0005 | <0.0005 |
2013 | 0.4 | 0.6 | <0.0005 | <0.0005 |
2014 | <0.2 | <0.2 | <0.0005 | <0.0005 |
2015 | 0.2 | 0.4 | <0.0005 | <0.0005 |
2016 | <0.8 | <0.8 | <0.0005 | <0.0005 |
2017 | <0.8 | <0.8 | <0.0005 | <0.0005 |
2018 | <0.7 | <0.7 | 0.0008 | 0.0008 |
2019 | <0.7 | <0.7 | <0.0005 | <0.0005 |
2020 | <0.7 | <0.7 | <0.0005 | <0.0005 |
2021 | <0.7 | <0.7 | <0.0005 | <0.0005 |
2022 | <0.7 | <0.7 | <0.0005 | 0.0006 |
1 HC (Health Canada). 2019. Guidelines for Canadian drinking water quality: Guidelines for Canadian Drinking Water Quality Guideline Technical Document - Uranium. Ottawa, Ontario. (Catalogue No. H144-13/12-2019E-PDF).
2 CCME (Canadian Council of Ministers of the Environment). 1999. Canadian Water Quality Guidelines for the Protection of Aquatic Life. Uranium.
3 HC (Health Canada). 2009. Guidelines for Canadian drinking water quality: Guideline technical document - radiological parameters. Radiation Protection Bureau, Healthy Environments and Consumer Safety Branch, Health Canada, Ottawa, Ontario. (Catalogue No. H128-1/10-614E-PDF).
Table 4: Annual average and maximum uranium values for Port Hope Harbour waters from 2006 to 2022. For comparison, uranium guideline values are 20 µg/L in drinking water1 and 15 µg/L for the protection of aquatic life.2
Sampling year | Uranium annual average (µg/L) | Uranium annual maximum (µg/L) |
---|---|---|
2006 | 5.5 | 16 |
2007 | 5.4 | 14 |
2008 | 6.5 | 21 |
2009 | 5.5 | 16 |
2010 | 4.4 | 21 |
2011 | 4.1 | 9.2 |
2012 | 3.7 | 10 |
2013 | 3.3 | 8.3 |
2014 | 3.3 | 7.6 |
2015 | 2.9 | 6.6 |
2016 | 2.6 | 10 |
2017 | 3.3 | 8.8 |
2018 | 5.2 | 31 |
2019 | 5.1 | 46 |
2020 | 5 | 12 |
2021 | 70* | 540* |
2022 | 120* | 500* |
1 HC (Health Canada). 2019. Guidelines for Canadian drinking water quality: Guidelines for Canadian Drinking Water Quality Guideline Technical Document - Uranium. Ottawa, Ontario. (Catalogue No. H144-13/12-2019E-PDF).
2 CCME (Canadian Council of Ministers of the Environment). 1999. Canadian Water Quality Guidelines for the Protection of Aquatic Life. Uranium.
* Production mechanical dredging commenced in the Port Hope Harbour in June 2021. Once contaminated sediment is removed from the harbour, water quality is predicted to improve. A robust monitoring program is being implemented by CNL to ensure the protection of the aquatic environment while dredging activities continue at the Port Hope Harbour. There are no direct or indirect exposure pathways for human receptors, and the area of impact is localized and does not extend into the Ganaraska River or Lake Ontario. Adverse effects associated with the concentrations detected are considered unlikely.
Table 5: Annual average tritium and gross beta values for the 2019–2022 monitoring period for near-field water supply plants. For comparison, the tritium drinking water guideline1 is 7,000 Bq/L, and the gross beta drinking water guideline1 is 1 Bq/L.
Nuclear power plant | Water supply plant | Tritium annual average (Bq/L) | Tritium annual maximum (Bq/L) | Gross beta annual average (Bq/L) | Gross beta annual maximum (Bq/L) |
---|---|---|---|---|---|
Bruce | Kincardine | 4.7 | 5.5 | 0.06 | 0.07 |
Bruce | Southampton | 11.2 | 12.4 | 0.08 | 0.13 |
Pickering | Ajax | 5.2 | 5.7 | 0.11 | 0.12 |
Pickering | F.J. Horgan | 4.0 | 4.6 | 0.11 | 0.12 |
Pickering | R.C. Harris | 3.6 | 4.3 | 0.11 | 0.11 |
Pickering | Whitby | 5.6 | 6.0 | 0.11 | 0.12 |
Darlington | Bowmanville | 4.9 | 5.2 | 0.11 | 0.11 |
Darlington | Newcastle | 4.5 | 4.8 | 0.11 | 0.11 |
Darlington | Oshawa | 6.5 | 6.7 | 0.11 | 0.12 |
1 HC (Health Canada). 2009. Guidelines for Canadian drinking water quality: Guideline technical document - radiological parameters. Radiation Protection Bureau, Healthy Environments and Consumer Safety Branch, Health Canada, Ottawa, Ontario. (Catalogue No. H128-1/10-614E-PDF).
Table 6: Annual average and maximum tritium and gross beta values for the 2019–2022 monitoring period for near-field lake surface water samples. For comparison, the tritium drinking water guideline1 is 7,000 Bq/L, and the gross beta drinking water guideline1 is 1 Bq/L.
Nuclear power plant | Location | Tritium annual average (Bq/L) | Tritium annual maximum (Bq/L) | Gross beta annual average (Bq/L) | Gross beta annual maximum (Bq/L) |
Bruce | Baie du Doré | 98.0 | 163 | 0.09 | 0.10 |
Bruce | Inverhuron Park | 15.2 | 20.9 | 0.09 | 0.10 |
Bruce | Scott Pt. Lake | 45.4 | 55.2 | 0.07 | 0.08 |
Pickering | Frenchman’s Bay | 32.4 | 39.5 | N/A2 | N/A2 |
Pickering | Beachfront Park | 22.0 | 25.8 | N/A2 | N/A2 |
Darlington | McLaughlin Bay | 14.9 | 22.9 | N/A2 | N/A2 |
Darlington | Courtice Road Beach | 6.1 | 6.8 | N/A2 | N/A2 |
1 HC (Health Canada). 2009. Guidelines for Canadian drinking water quality: Guideline technical document - radiological parameters. Radiation Protection Bureau, Healthy Environments and Consumer Safety Branch, Health Canada, Ottawa, Ontario. (Catalogue No. H128-1/10-614E-PDF).
2 Pickering and Darlington do not monitor gross beta in surface waters.
Table 7: Annual average (Avg) and maximum (Max) radionuclide activity levels (Bq/kg fresh weight) for white suckers from exposure locations over the assessment period (2019–2022). For comparison, the CNSC screening levels1 (Bq/kg fresh weight) are 488,000 for Tritium (H-3), 710 for Cesium-134 (Cs-134), 1,040 for Cesium-137 (Cs-137), 16,800 for Carbon-14 (C-14), 1,350 for Cobalt-60 (Co-60), and 1,020 for Potassium-40 (K-40).
Nuclear power plant | H-3 Avg | H-3 Max | Cs-134 Avg | Cs-134 Max | Cs-137 Avg | Cs-137 Max | C-14 Avg | C-14 Max | Co-60 Avg | Co-60 Max | K-40 Avg | K-40 Max |
---|---|---|---|---|---|---|---|---|---|---|---|---|
Bruce | 20.0 | 41.8 | <Lc2 | <Lc2 | 0.18 | 0.20 | 229 | 241 | 0.04 | 0.1 | 112* | 115* |
Pickering | 5.4 | 6.0 | <0.1 | <0.1 | <0.1 | <0.1 | 269 | 275 | <0.1 | <0.1 | 116 | 121 |
Darlington | 4.6 | 5.4 | <0.1 | <0.1 | <0.1 | <0.1 | 249 | 265 | <0.1 | <0.1 | 124 | 129 |
1 Visit Appendix A in CNSC 2018 for screening level derivation.
2 Bruce Power reports levels indistinguishable from background as <Lc (below critical level).
* Bruce Power removed Potassium-40 from their gamma spectroscopy results in 2021. Therefore, the results here are averaged from 2019–2020.
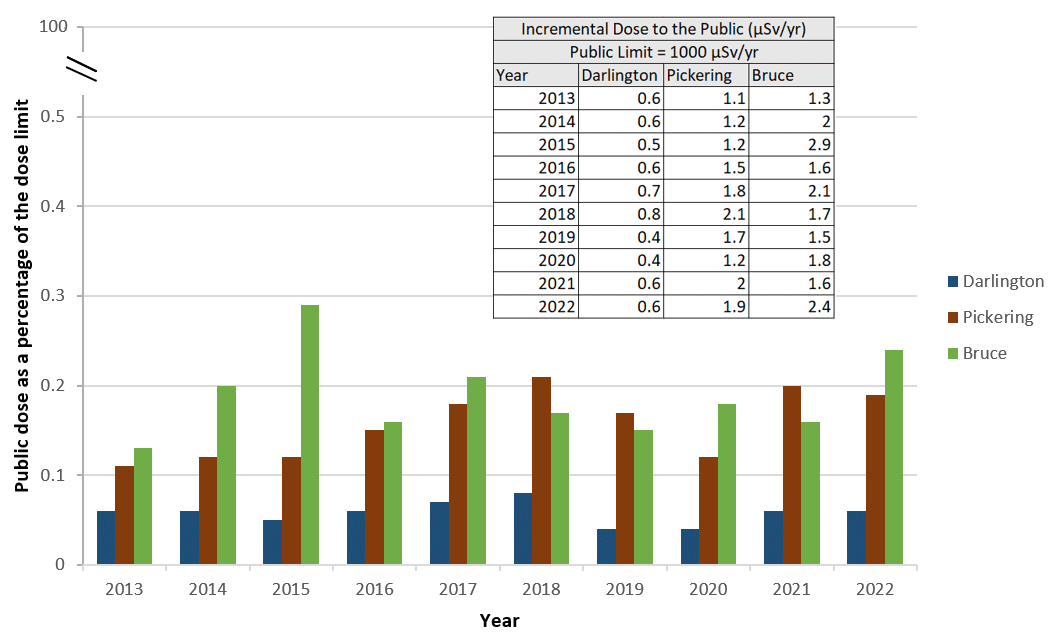
Figure 13: Maximum annual incremental dose to the public for CANDU reactor sites on the Great Lakes for the assessment period (2013–2022). Presented graphically as a percentage of the public dose limit with insert table providing the dose in µSv/year. Note: 1 mSv/year = 1000 µSv/year.
Related Links
Page details
- Date modified: